When something is not right, signals of some sort are sent out. While many are obvious - an engine gone dead, water flooding a basement, flames protruding from a rooftop - others are more subtle: the slim crack in a dam before its collapse, the ray of sun about to set off a forest fire, the faint smell of gas before an explosion. Humans have developed ways of sensing and measuring these more discreet signals in the hope of predicting and preventing catastrophes. On a far smaller scale, cells too have ways of sensing something that has gone wrong. This can be in the form of entities such as molecules - either foreign or synthesized by the cell itself in situations of stress - which trigger off defence mechanisms. It can also be something far less tangible, such as patterns. Patterns? Imagine small weaving inaccuracies in cloth for example. This is not unlike unusual conformations which may arise in stretches of DNA or RNA (known as Z-DNA or Z-RNA) when cells are dealing with stress such as viral infection for instance. While, in the 17th Century, Antonie van Leeuwenhoek perfected the magnifying lens to spot stray stitches in cloth, over time cells have perfected their own lens to spy Z-DNA and Z-RNA: a protein known as ZBP1.
In the 1970s, barely twenty years after the double-helical structure of DNA had been discovered, while observing X-ray images, the American biochemist Robert Wells noticed a peculiar twist in the backbone structure of a synthetic double-stranded DNA polymer. Instead of the usual smooth helical right-handed structure of DNA, it looked more like a zig zag - hence the name that it was later given: Z-DNA - despite the fact that the base-pairs and the sugar backbone were identical to those in the more classical DNA (also known as B-DNA). The discovery begged the question: does Z-DNA actually exist in live cells? And if so, does it have any biological relevance? The answer to the first question turned out to be: yes. The second has been subject to much debate but, almost 50 years later, it is now believed that stretches of DNA can flip to the left-handed Z conformation to relieve torsional strain during transcription - in the way a wet towel spontaneously unwinds when it has been wrung out tightly. Z-DNA would therefore be yet another mechanism used by cells in the process of decoding genetic information.
So short stretches of Z-DNA can be observed close to where transcription occurs in cells. But what is happening on the molecular scale? What is it that gives Z-DNA its zig zag configuration? Each Z-DNA stretch begins and ends with what has been coined a B-Z junction - where one base pair has snapped to relieve strain. The resulting end-bases stick out on either side of the DNA structure instead of facing in. In between the two junctions, the double helix is still held together by the traditional Watson Crick base pairs, but instead of the sugar backbone twisting smoothly as it traces major and minor grooves, the grooves traced by the backbone of Z-DNA are of similar width while the orientation of the base pairs relative to the sugar backbone are uncharacteristic. As a consequence, the backbone of flipped DNA adopts a zig zag conformation.
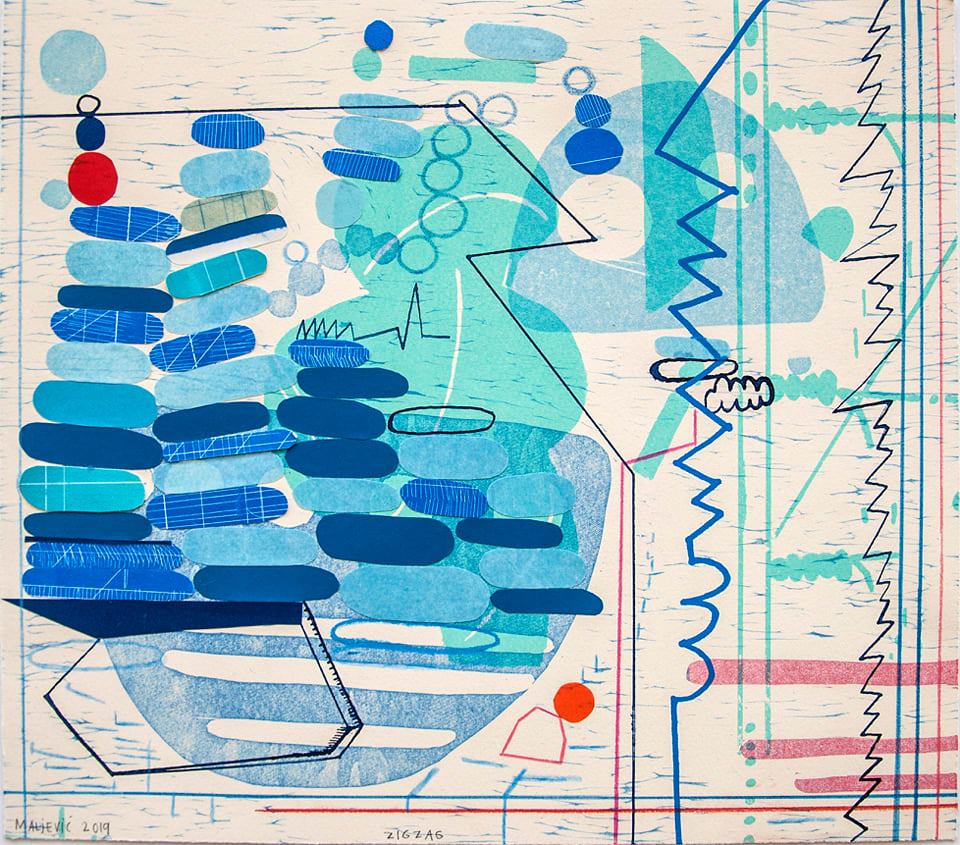
Courtesy of the artist
As the years rolled by and research did too, it soon became apparent that double-stranded RNA could also adopt the Z conformation. This has been observed in the course of viral infection - such as influenza A virus (IAV) for example - during which Z-RNAs are produced. These stretches of Z-RNA are not part of the viral genome but seem to be by-products of the virus as it multiplies. Their formation provide telltale signals - albeit unintentional on behalf of the virus - that something is not right and infected cells are swift to pick them up. This is where the so-called Z-DNA Binding Protein, or ZBP1, comes into action. Human ZBP1 is a pathogen 'pattern' sensor in that it is able to recognize and bind to Z-RNAs produced during IAV infection. Once bound, specific pathways are activated, ultimately leading to host cell death and inflammation - both of which are distinct immune responses although interconnected and mutually regulated. Host cell death may sound counterintuitive when a host is fighting off infection, but it is one way - as long as it is controlled - of ensuring that viral replication is stunted.
How does ZBP1 know when to recognize stretches of Z-RNA in a host cell? ZBP1 seems to be expressed when cells are undergoing stress. The protein is found mainly in a cell's cytoplasm within what are known as stress granules and processing bodies. These entities form when, under stressful conditions, a cell decides to defer translation or perhaps even resume it. RNA molecules stored within stalled translation complexes are held in stress granules, and if a cell decides to stop translation altogether, the contents of the stress granules are destroyed thanks to the processing bodies. Following IAV infection, however, ZBP1 rapidly accumulates in the host cell's nucleus where the replicating RNA virus is generating Z-RNAs.
How exactly ZBP1 senses Z-RNA has still to be understood, but two domains in its sequence are important: Zα and Zβ, which are similar to one another and situated at the protein's amino-terminus. These domains are now known to bind specifically to Z-RNA or to Z-DNA; this is explained by the fact that ZBP1 binds to the sugar backbone rather than to the bases. As a consequence, ZBP1 is not specific to a nucleotide-base sequence but rather to the left-handed conformation of Z-RNA, or indeed of Z-DNA. Downstream of Zα and Zβ are two other domains known as RHIM domains, which are necessary to complete the immune response. Once bound to Z-RNA and by way of the RHIM domains, ZBP1 activates the beginning of a cascade ultimately leading to cell death by the disruption of the nuclear envelope and leakage of the cell's DNA into the cytosol.
The system is a very subtle way of sensing danger and launching an immune response. Though human ZBP1 is known to bind to Z-RNA during IAV infection - which is unique in that IAV is an RNA virus which replicates in the host cell's nucleus - ZBP1 can also bind to Z-DNA, and Nature has graced life with both DNA and RNA viruses. Besides sensing viral infection, ZBP1 seems to be associated with the onset and progression of certain cancers, as well as several autoimmune diseases. This does not really come as a surprise since, if ZBP1 were to be defective, cell death could fire ahead unbridled subsequently causing severe damage to the host, despite the fact that a virus has been wiped out. Currently, therapeutic strategies for targeting Z-DNA and the proteins that bind to it to manipulate the expression of local genes is being considered. Still, though ZBP1 has participated in demonstrating the biological relevance of Z-DNA, it continues to puzzle scientists. How is it activated? But more intriguingly, perhaps, how is a protein capable of sensing a change of pattern in DNA structure?